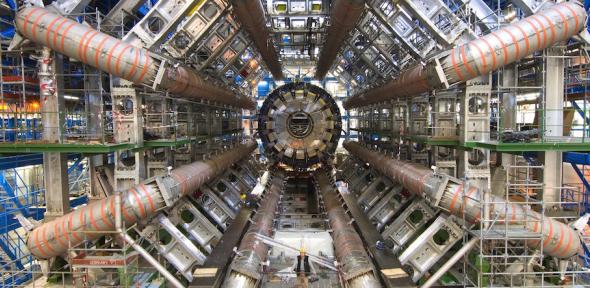
The interplay between theoretical and experimental physics came to world-wide attention in recent years with the discovery of the Higgs boson at the Large Hadron Collider, fifty years after it was predicted by theoretical physicists.
Maria Ubiali, University Lecturer in the Department of Applied Mathematics and Theoretical Physics, works at this interface between theory and experiment. She is a phenomenologist, working on the observable consequences of the laws of nature, and in particular the laws of particle physics as described by the mathematical theory called the Standard Model.
"What I really do is to formulate theoretical predictions, to give numbers to the experimentalists," says Ubiali. "They verify what I give them to see if the Standard Model is the law that correctly describes nature, or if there is something more than that."
What can we predict?
A good example of the interplay between theory and experiment is our evolution of the understanding of the proton. When protons were discovered a century ago, scientists thought they were elementary, indivisible particles. In the 1960s it was realised that protons were actually built of three smaller particles, which we now call quarks, bound together by some force carrying particles called gluons. And today our picture of protons is even more complicated. "There is an interesting life inside the proton," says Ubiali. "Inside this tiny radius of the proton quarks keep creating and annihilating in pairs, quarks and antiquarks, gluons being radiated all the time off the quarks and antiquarks."
At high energies (when quark/antiquark pairs are more likely to pop in and out of existence) you might think of a proton as more like a swarm of particles: three quarks, and a crowd of gluons and quark/antiquark pairs. The structure of the proton is then described in terms of statistical distributions for how the properties of the proton, such as its momentum, are distributed across the swarm.
Machine learning
As a theorist I'm really interested to see what is beyond the Standard Model. I’m particularly interested in the paradigm shift we are living in.Maria Ubiali
Theoretical predictions for processes involving elementary particles such that electrons or photons can be determined from the standard model by solving the relevant equations. "What is interesting about the structure of the proton is that it can't be determined by using first principles," says Ubiali. For the mathematical description of the protons, called quantum chromodynamics (QCD), the equations cannot be completely solved. "That's why we need experimental data to infer the structure of the proton in terms of its elementary constituents."
And here is where machine learning can help. There is a huge amount of data from the LHC and other experiments from which we can infer the structure of the proton. Ubiali and her colleagues start with an unbiased mathematical description of the structure, then find patterns in these large data sets to fine tune the parameters of the mathematical description.
"That's how we apply machine learning, so we have a much better determination of the [proton's structure]," explains Ubiali. And this data-driven description not only gives the best prediction of the structure, but also the uncertainty associated with this best prediction. "We have more realistic estimates of the uncertainty, of what we still don't know."
Beyond the Standard Model
"The success of the Standard Model is extraordinary," says Ubiali. Recent experiments that have compared the Standard Model's predictions to experimental data show an amazing agreement over a wide range of scales (from 10-4 to 1011 pico barns – a measurement of area). However we know that the Standard Model cannot be a complete description of nature as there are many mysteries – such as why neutrinos have mass and what is dark matter – that the Standard Model cannot solve.
Ubiali says that part of her work involves stretching the Standard Model to the maximum precision possible in order to experimentally observe any deviations from the predictions. And then she also works on some particular models that go beyond the Standard Model.
"As a theorist I'm really interested to see what is beyond the Standard Model. I’m particularly interested in the paradigm shift we are living in. When the LHC was turned on we were all expecting to see some spectacular deviation from our predictions because we would reach the energy [of the collisions] when something would break down. But that didn't happen."
"This could be a source of depression, or a source of excitement. For me it is a source of excitement - it means that nature will give us some more subtle hints about deviations."